Materials Refining
Work Stream 3
During operation, the active materials within a battery undergo multiple degradation processes like a loss in Li inventory, particle cracking and transition metal dissolution. Once materials are extracted from the cell and separated in WS2, processes must be employed that can restore active materials to a pristine condition to be reused and remanufactured into new cells in WS4.
Reconditioning the active materials for reuse through short-loop or direct recycling (WP3.5 and WP3.6) rather than converting them to raw materials such as metals or metal salts reduces process steps and costs and provides a pathway to greener recycling processes.
The state-of-art of battery chemistries used are evolving, meaning materials currently entering the recycling chain are mainly of a redundant technology and consequently of low value.
In WP3.3 we are developing processes to upcycle active materials from older generation batteries to more commercially relevant materials. For example, graphite anodes can be converted to graphite-SiOx anodes or LiMn2O4 cathode materials can be converted to higher capacity Ni-doped spinels.
During ReLiB previous phases processes were developed for refining anode and cathode materials. Part of this work has involved the publication of a patent on selective leaching and direct recycling. These processes have been applied to recycle, regenerate and upcycle materials. Further work is required to expand these studies to a range of cathode and anode materials and different battery chemistries to cover Na-ion and solid-state batteries (WP3.1, WP3.3, WP3.5 and WP3.6).
Applying these processes to next generation materials will help future-proof processes. In combination with hydrometallurgical leaching, bioseparation processes have been developed during ReLiB previous phases that can recover critical materials from waste leachates (WP3.4). Further development is required, including scale up of processes, which has already begun in collaboration with the Industrial Biotechnology Innovation Centre (IBioIC) based in Glasgow.
The ReLiB project aims to recycle and reuse all components of batteries so in addition to the development of recycling processes for Li-ion battery electrodes, WP3.2 investigates electrolyte recovery and regeneration. Development of Lirecovery processes from electrodes and electrolyte are currently being developed and will be further expanded and optimized during ReLiB phase 2.
WP3.1
Leaching & Extraction
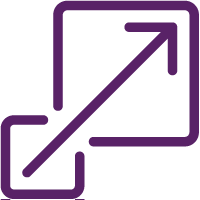
Scope
Electrode material (from WS2) will be characterized and appropriate leaching routes determined to extract component metals or compounds into solution, to provide both liquid/solid fractions for WP3.3, 3.4 & 3.5.
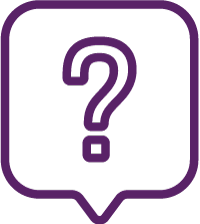
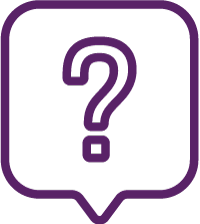
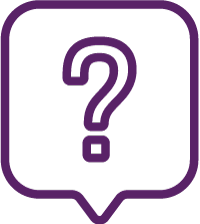
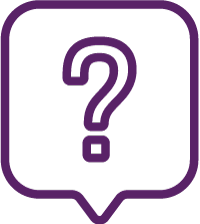
What is Involved
Complement work in WS2 with chemical (leaching/extraction) techniques to separate components and deliver purer waste streams and/or residual solids suitable for direct recycling.
Build on ReLiB previous phases patented technology developing separation processes that illustrate selectivity for components e.g. selective Li leaching from Li4Ti5O12 anodes, selective dissolution of one electrode material from a blended electrode or mixture of electrodes (such as used in new dual chemistry battery packs), or selective removal of coating layers.
Through fundamental understanding of hydrometallurgical processes, we can exploit differences in solvation of metals/materials to produce higher value fractions for use in WP3.3, 3.4 & 3.5.
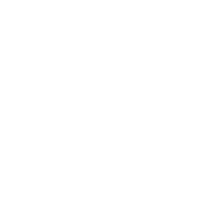
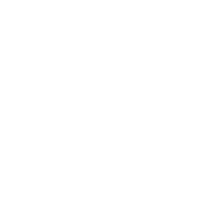
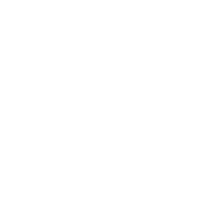
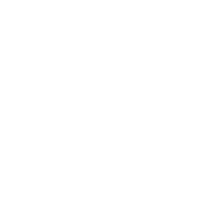
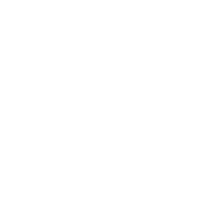
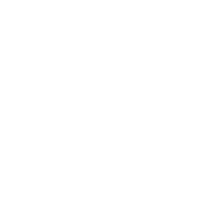
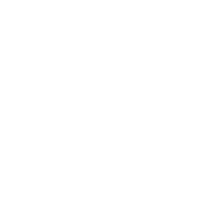
Objectives
- From materials characterization information on the components of LIBs and future technologies (SSBs, NIBs) provided by WP2, derive appropriate leaching/ metal extraction approaches to deliver high purity fractions for WP3.3, 3.4, 3.5
- Extend selective leaching approaches to other electrode mixtures, including separation of black mass (to complement WP2) and deal with shredded dual chemistry battery packs
- Extend selective leaching approaches to electrode materials with surface coatings, and Li recovery from anode materials, including LTO
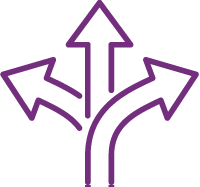
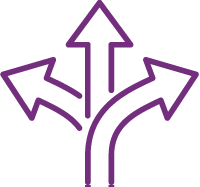
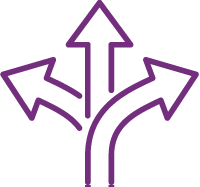
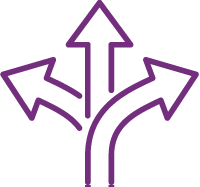
Pathways to Impact
M1–12: Extend selective leaching approaches to a range of electrode mixtures, as well as selective Li recovery processes from a range of cathode and anode materials.
M6–18: Investigation of routes for selective removal of coating layers to allow more facile relithiation / repair of electrode materials.
M18-24: Scale up of most promising approaches.
In previous phases IP has been protected and is being commercialized through the ReLiB TPIP.
WP3.2
Electrolyte Recovery & Regeneration
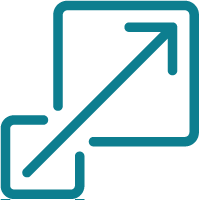
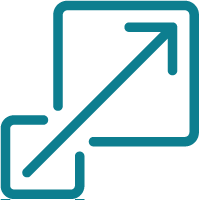
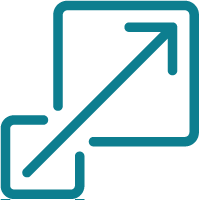
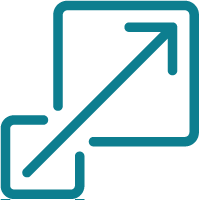
Scope
This package focuses on process development of electrolyte solvents and conductive lithium salts recovery, and analysis of ingredients using HPLC, GCMS and IC. Preliminary work on recovery process includes supercritical CO2 extraction of electrolyte solvents and extraction kinetics, and development of HPLC methods for analysis of Li and other active ingredients. Solvents to extract electrolyte and evaluate recovered electrolytes and Li after refining and regeneration will be investigated.
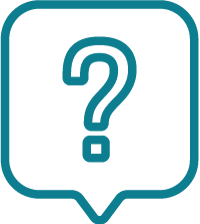
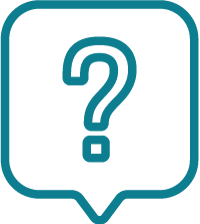
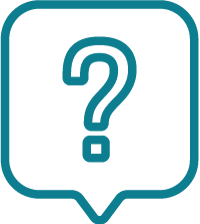
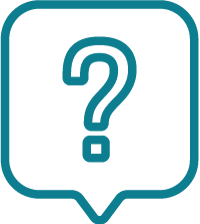
What is Involved
Electrolyte accounts for approximately 4% of the total cost of LIBs, but inappropriate treatment during recycling may cause chemical hazards, material inefficiency and loss, and environmental pollution. CO2 with or without co-solvents have been demonstrated to be effective in extracting both carbonate electrolyte solvents and conductive lithium salts (such as LiPF6 and LiBF4). Owing to complex chemical reactions that occur during the use of LIBs, the extracted electrolytes include various impurities and these must be removed before reuse.
Successful analysis of the extracted ingredients is essential for the success of electrolyte recovery and regeneration. HPLC, GCMS and IC (ion chromatograph) have been used for analysis of salts and electrolyte ingredients. After identification of the impurities, refining methods need to be developed to remove the impurities.
Efficient process development requires both extraction and refining processes based on the extraction kinetics, with improved understanding of the hydrodynamics, and improved heat and mass transfer methods.
Electrolyte recovery and regeneration is essential both for avoiding chemical hazards during recycling and for enabling the reuse of the electrolyte solvents and lithium salts.
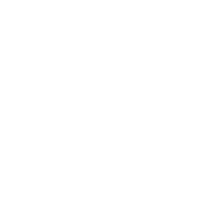
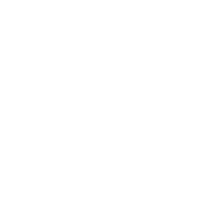
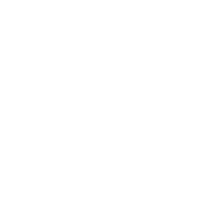
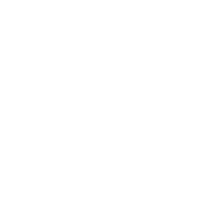
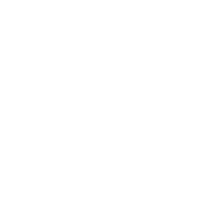
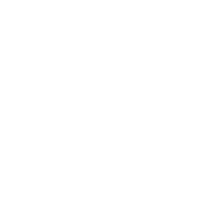
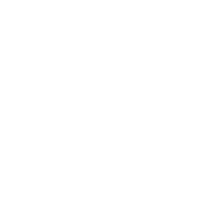
Objectives
- Analyse the electrolyte solvents and conductive lithium salts in the EoL and QC-failed batteries; use HPLC and GCMS for analysis of the chemical changes and to identify impurities
- Identify nature and extent of electrolyte aging at different cell life stages
- Develop routes for recovery of electrolyte and Li from QC-failed and EOL cells using CO2, deep eutectics and selected co-solvents
- Separate impurities from the extracted electrolyte solvents; characterize and assess recovered electrolyte for cell or other applications
- Undertake process development for extraction methods including extraction kinetics, hydrodynamics and transport phenomena
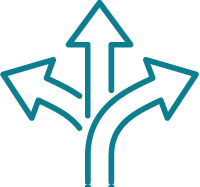
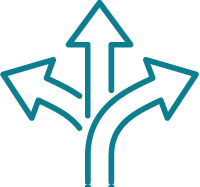
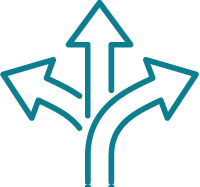
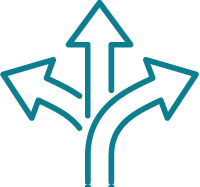
Pathways to Impact
For maximum impact – and maximum efficiency it will be necessary to develop and integrate electrolyte recovery with other parts of the recovery process e.g. delamination, PVDF removal.
WP3.3
Active Material Upcycling
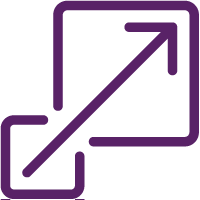
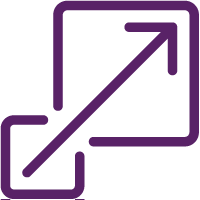
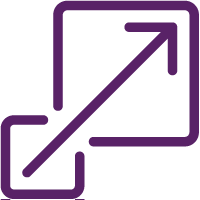
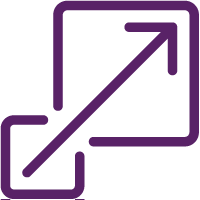
Scope
A challenge in the battery recycling area is the changing chemistries that are used in cells so when vehicles reach the end of their life and the batteries go to be recycled, there is a likelihood that the cell chemistry may now be obsolete. If the advantages of short loop/direct recycling are to be realized, there is therefore a need to develop low cost methods for the use of material recovered from WP3.1 to make new higher performing materials (upcycling).
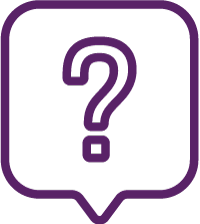
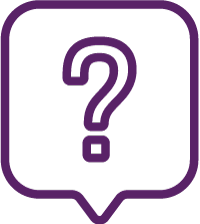
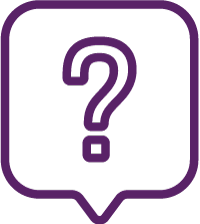
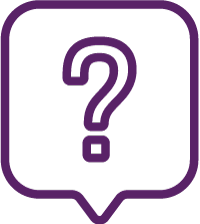
What is Involved
Upcycle recovered material from EoL cells into current generation battery materials (both cathodes and anodes) or future electrode material targets (linking with FI cathodes projects – key ReLiB CoIs currently also on CATMAT), ensuring all components (including low value) can be reutilised.
Characterise the performance of remanufactured electrode materials and compare to that of materials prepared from pristine precursors.
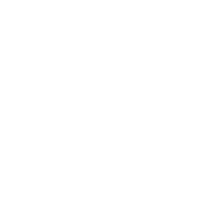
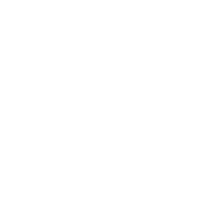
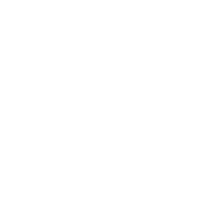
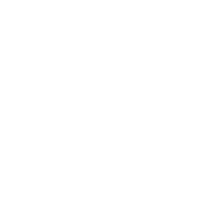
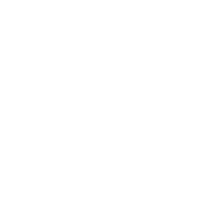
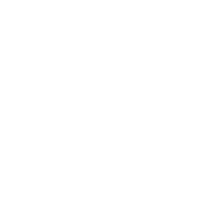
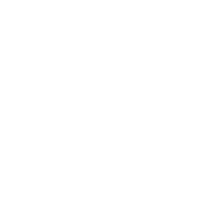
Objectives
- Optimize the upcycling of low Ni content NMCs to current generation high Ni NMCs, including surface stabilization to improve stability
- Take low value (e.g. LMO) materials and upcycle to high voltage Ni doped LMO and Na ion battery cathodes (with Li recovery in conjunction with WP3.1) to reutilize the Mn
- Future-proof our recycling processes by extending the work to investigate next generation cathode materials, such as core-shell materials, higher capacity Li-rich layered oxides, and disordered rock salt compounds
- Upcycle anode materials e.g. graphite to graphite–SiOx; Li4Ti5O12 to TiNb2O7 (with Li recovery)
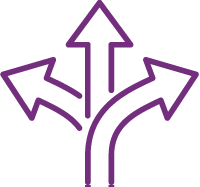
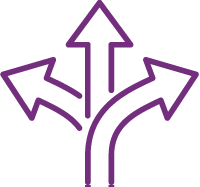
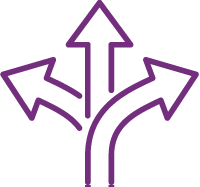
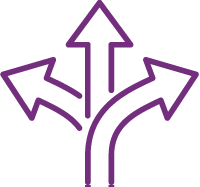
Pathways to Impact
M1–12: Optimize the upcycling of EoL materials to high Ni NMCs and explore surface stabilization; evaluate graphite upcycling to graphite–SiOx.
M6–24: Extend cathode work to Na ion battery materials, and next generation Li ion battery materials; extend anode work to oxide anode materials. In previous phases IP has been protected and may be commercialised through the ReLiB Technology Platform Implementation Plan.
WP3.4
Biological Recovery
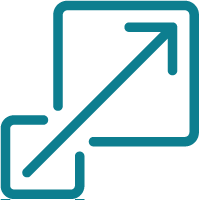
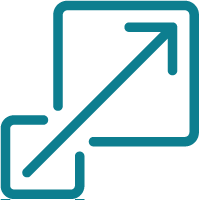
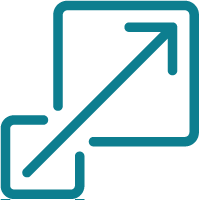
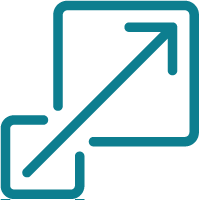
Scope
This work package focuses on the design and engineering of bacteria for bioseparation of metals to provide a biological solution for low-cost metal reclamation from low-concentration aqueous waste, offering the ability to separate similar transition metals such as Co and Ni. It aims to progress production of up-cycled nanoparticles suitable for remanufacturing from real world leachates.
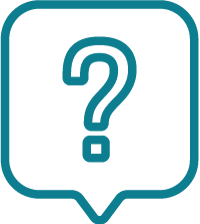
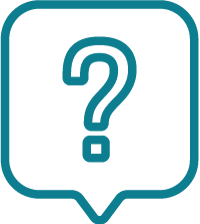
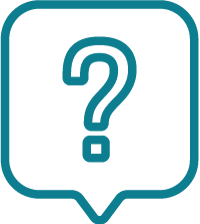
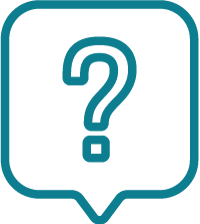
What is Involved
The main focus of this work package is to build upon our current manganese bioseparation process, applying it to wastes from a wide range of battery types (not only LIBs) and hydrometallurgical methods. The use of selective metal bioseparation methods offers potential for the development of greener alternatives to current recycling methods, based on the principles of green chemistry (aqueous solutions, low reaction temperatures) with scope for reducing CO2 emissions and waste production.
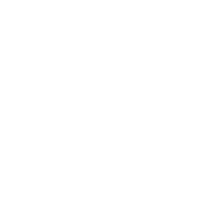
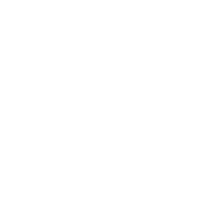
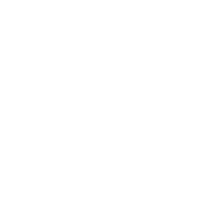
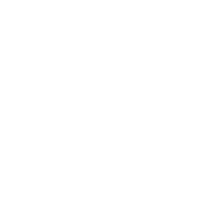
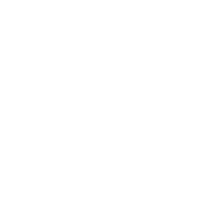
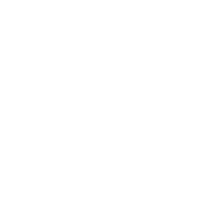
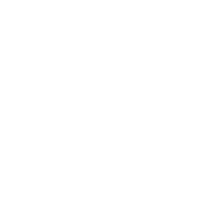
Objectives
- Scale processes using industrial leachates for Mn bioseparation to semi-pilot scale (30L)
- Synthesize metallic nanoparticles for remanufacturing precursors or other applications outside of batteries
- Evaluate the potential for engineered bacteria to synthesize battery precursor materials directly without further processing
- Investigate cell-free bacterial systems
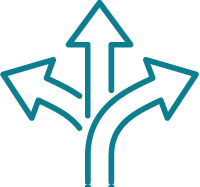
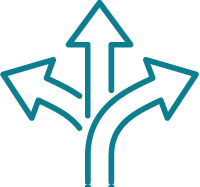
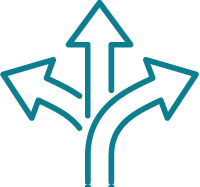
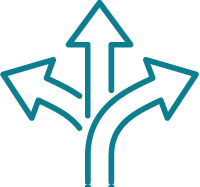
Pathways to Impact
Scale up activities begun in collaboration with the Industrial Biotechnology Innovation Centre (IBioIC) in Glasgow will continue.
WP3.5
Short-loop Recycling
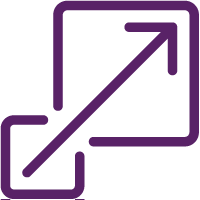
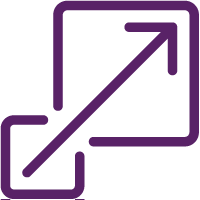
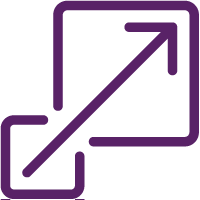
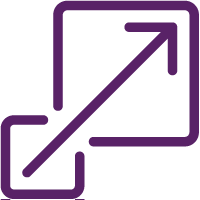
Scope
Short-loop recycling involves reusing and regenerating battery materials using a minimal number of steps/processes rather than breaking them down into raw materials such as metal salts.
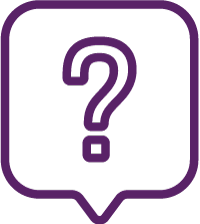
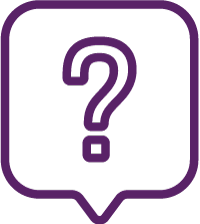
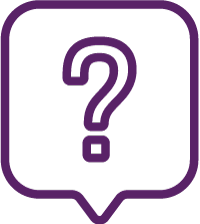
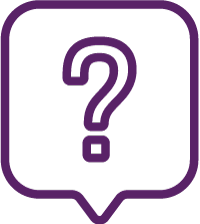
What is Involved
Development of selective leaching routes to separate complex mixtures of active materials (e.g. LFP from NMC/NCA, black mass) and regenerate electrode materials suitable for remanufacturing.
Complements work in WS2, WS3.1, WS3.3 and WS3.6 while also expanding on methods developed for larger scale regeneration of electrode materials.
From characterization of the materials and performance of electrodes developed through short-loop recycling in WP3.5, evaluation of performance can be developed in WP4.
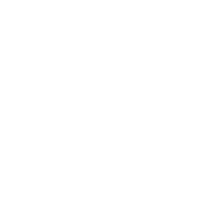
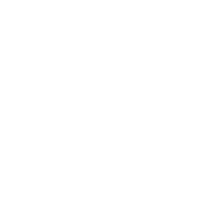
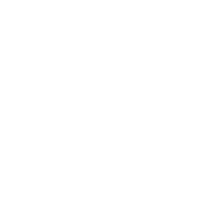
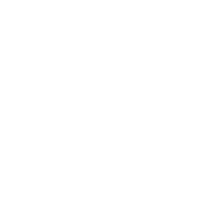
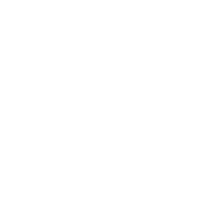
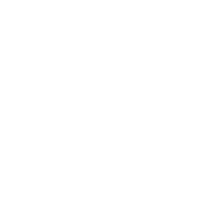
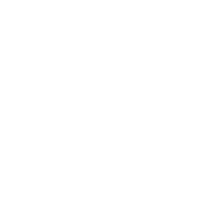
Objectives
- Expand on work from existing selective leaching patent to demonstrate short-loop recycling processes at scale
- Demonstrate selective leaching and short-loop recycling processes on other mixtures and materials (e.g. LFP, Na-ion batteries, black mass)
- Demonstrate short-loop recycling processes for next generation Li-ion, Na-ion and solid-state battery materials
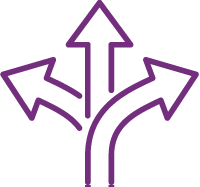
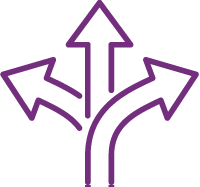
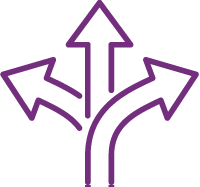
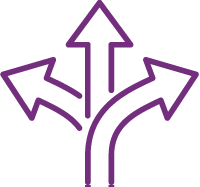
Pathways to Impact
Extend selective leaching approaches to a range of electrode mixtures and develop short-loop recycling processes for next generation materials.
Scale-up of most promising approaches and existing patented work to demonstrate commercial viability (with WS4 and the ReLiB TPIP).
WP3.6
Direct Recycling
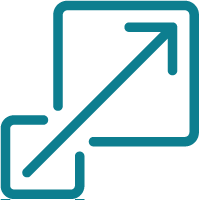
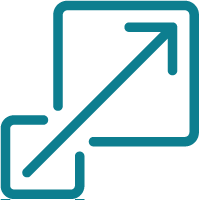
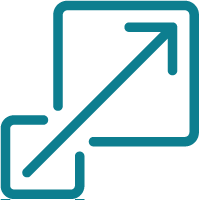
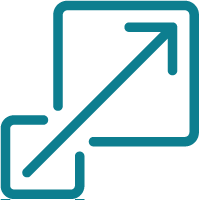
Scope
Direct recycling involves the repair of materials that are recovered from the recycling process, such that they can be put back into a cell and exhibit a comparable performance to the pristine material.
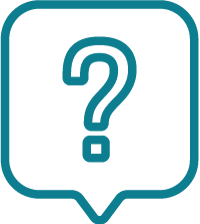
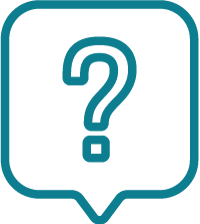
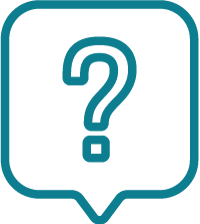
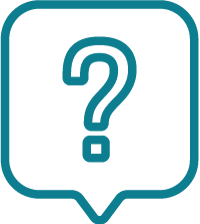
What is Involved
Characterisation of initial recovered material to determine the nature of degradation, e.g. cathode: loss of Li inventory, particle cracking, DRS layers.
Development of routes to overcome these degradation factors, including solvothermal, elevated temperature solid state routes and lower energy milling (e.g. acoustic) approaches; the low T approaches will be utilized to avoid side reactions/inter-diffusion of coatings at elevated temperatures.
Characterise the performance of these remanufactured electrode materials and compare the performance to comparable materials prepared using pristine precursors. For some anode materials, e.g. TiNb2O7 there is the potential that the directly recycled material may illustrate better performance as the irreversible Li present may reduce/eliminate first cycle irreversible capacity loss.
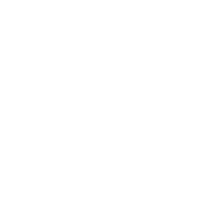
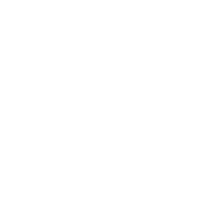
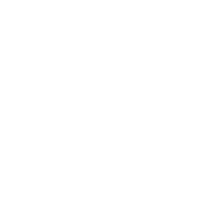
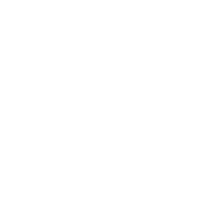
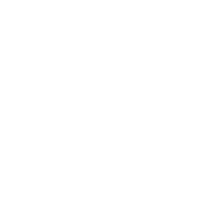
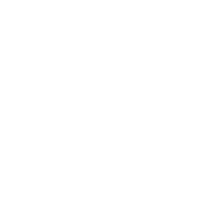
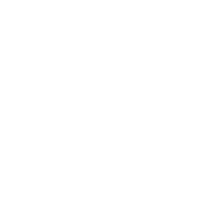
Objectives
- Develop routes for the direct recycling of cathode materials, including high Ni content materials which have protective coatings
- Extend work illustrating the potential for graphite reuse to direct recycling of higher capacity graphite–SiOx anodes
- Develop and illustrate the potential of routes for the direct recycling of high power anodes, such as Nb-based oxides
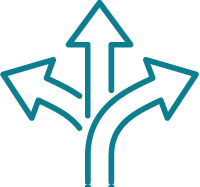
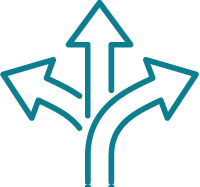
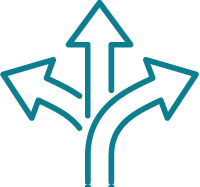
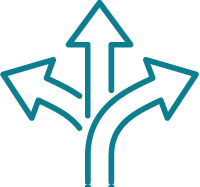
Pathways to Impact
M1–12: Evaluate the performance of directly recycled anode materials (graphite-SiOx and Nb-based oxides).
M6–15: Develop routes to directly recycle high Ni NMCs.
M12–24: Evaluate the effect of coatings on the recycling process and develop routes to overcome issues.